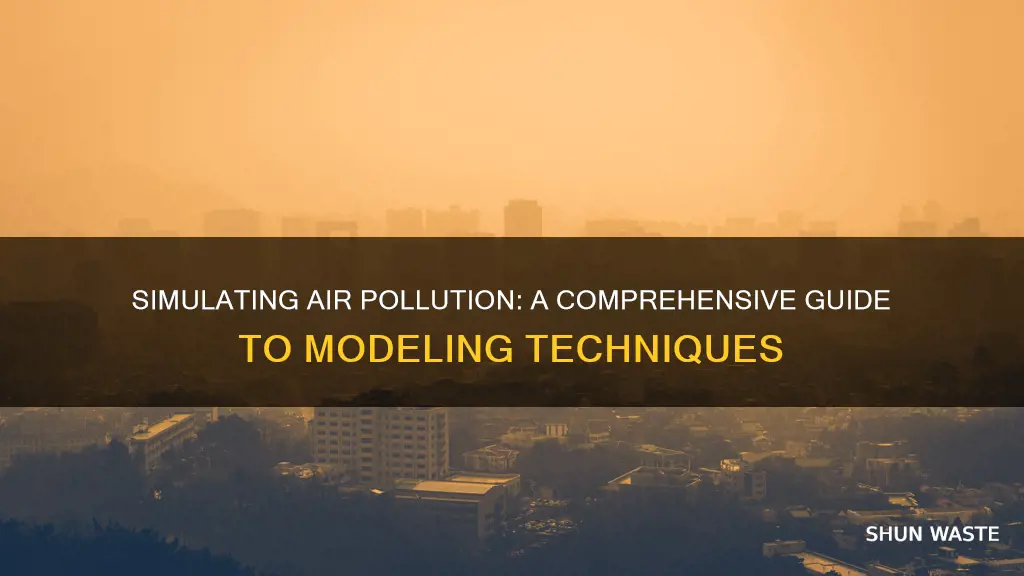
Air pollution is a pressing issue, and understanding its impact is crucial for developing effective strategies to mitigate it. To this end, various methods are employed to simulate air pollution and gain insights into its behaviour and consequences. These simulations play a pivotal role in air quality management and policy formulation. One prominent approach is the use of mathematical and numerical techniques to model the dispersion and chemical reactions of pollutants in the atmosphere. Additionally, wind tunnels and scaled models are utilised to replicate the spread of harmful airborne materials in urban and industrial areas. The SIM-air family of tools offers interactive models that leverage available information to enhance urban air quality management. Furthermore, software packages like IAQX, a Windows-based simulation tool, aid in analysing indoor air quality and estimating exposure to pollutants. These diverse simulation techniques provide valuable tools for assessing and addressing the challenges posed by air pollution.
Characteristics | Values |
---|---|
Tools | SIM-air family of tools, Simulation Tool Kit for Indoor Air Quality and Inhalation Exposure (IAQX) |
Objective | Utilize the best available information on all the known sources of air pollution to support integrated air quality management |
Applications | Air Pollution knowledge Assessments (APnA) city program |
Simulation Techniques | Mathematical and numerical techniques, miniature models, wind tunnels |
Compatibility | Windows XP, 7, and 8 |
What You'll Learn
Using a wind tunnel to simulate air pollution
Wind tunnels are a useful tool for simulating air pollution. They are classified by the orientation of airflow in the test section with respect to gravity. Most wind tunnels are oriented horizontally, but vertical wind tunnels are also used to balance gravity with drag instead of lift. These vertical tunnels have become popular for recreational skydiving simulations.
Wind tunnels are also classified by their main use. For example, those used for ground vehicles like cars and trucks have different floor aerodynamics, from stationary floors to full moving floors. Aeronautical wind tunnels are subcategorized by the Reynolds number, a parameter that describes all fluid flow situations, including the shape of flow patterns and the onset of turbulence. Osborne Reynolds, an Englishman from the University of Manchester, demonstrated that the airflow pattern over a scale model would be the same for a full-scale vehicle if the Reynolds number were the same.
There are several ways to simulate a high Reynolds number, as it is impractical to obtain a full-scale Reynolds number with a full-scale vehicle. These methods include using pressurized tunnels, heavy gas tunnels, cryogenic tunnels, and high-altitude tunnels. Wind tunnel test sections can range in size from less than a foot across to over 100 feet, with air speeds varying from a light breeze to hypersonic.
At the EPA's meteorological wind tunnel facility in North Carolina, researchers use scaled models to simulate the spread of harmful airborne material released in urban and industrial settings. They can control variables such as wind direction, source locations, and the amount of pollutant. The scientists can also generate different amounts of turbulence with triangular spires and roughness elements on the tunnel floor to simulate landscape features. The models used in the wind tunnel are designed and handmade by the researchers, typically using wood or foam.
How Organizations Are Fighting Air Pollution
You may want to see also
Mathematical and numerical air quality models
Air quality models employ mathematical and numerical techniques to replicate the chemical and physical processes that influence the dispersion and reactions of air pollutants in the atmosphere. Meteorological data and source information, such as emission rates and stack height, are used as inputs for these models. They are designed to characterise primary pollutants directly emitted into the atmosphere and, occasionally, secondary pollutants formed through intricate atmospheric chemical reactions.
Mathematical models, such as atmospheric diffusion models, are used to estimate air quality by describing the dispersion of pollutants like sulfur dioxide. These models can be applied to various scenarios, such as elevated sources of pollution or urban heat islands, to understand their impact on air quality. For instance, the removal model proposed by U. Arora, S. Gakkhar, and R. S. Gupta in 1991 is suitable for air pollutants emitted from elevated sources.
Numerical simulations also play a crucial role in air pollution emission control near industrial zones. S. A. Konglok and N. Pochai developed a three-dimensional air quality model that considers variations in atmospheric stability classes and wind velocities using the fractional step method. This numerical approach allows for more precise calculations and predictions of air pollution dispersion.
Additionally, the SIM-air family of tools, or "Simple Interactive Models for better air quality," was developed to utilise the best available information on all known sources of air pollution to support integrated air quality management. These models can estimate emissions and simulate interactions between emissions, pollution dispersion, impacts, and management options. The first integrated version of SIM-air was built in 2002 using hardwired matrix multiplications for PM2.5 pollution analysis, and the current version offers more flexibility in testing management options and exploring new MS Excel functions for larger domains and multiple sectors.
Hazardous Waste and Air Pollution: A Toxic Mix
You may want to see also
Indoor air quality simulation software
Several indoor air quality simulation software packages have been developed to estimate inhalation exposure to indoor air pollutants. This is an essential part of multi-pathway exposure assessment, given that most people spend a large portion of their time indoors.
One such software is the Simulation Tool Kit for Indoor Air Quality and Inhalation Exposure (IAQX), a Microsoft Windows-based indoor air quality (IAQ) simulation package. It consists of a general-purpose simulation program and a series of stand-alone, special-purpose programs. The general-purpose program performs multi-zone, multi-pollutant simulations and allows gas-phase chemical reactions. It can also estimate adequate ventilation rates based on user-provided air quality criteria. The special-purpose programs contain more complex mass transfer models, including models for predicting volatile organic compound (VOC) emissions from solvent-based indoor coating materials. IAQX is designed for advanced users with experience in exposure estimation, pollution control, risk assessment, and risk management.
Another software package is STKi, which is also a Windows-based IAQ simulation software package. It consists of a general-purpose simulation program and four stand-alone, special-purpose programs. The general-purpose program performs multi-zone, multi-pollutant simulations and allows gas-phase chemical reactions. It has a large collection of models for sources, sinks, and air filters/cleaners, enabling simulations for a wide range of indoor air pollution scenarios.
Other Windows-based IAQ simulation programs include RISK (Sparks, 1996), MEDB-IAQ (Zhang et al., 1999), and MCCEM (Koontz and Wilkes, 1999). Additionally, there is a web-based tool called FaTIMA for modelling the Fate and Transport of Indoor Microbiological Aerosols. It is a recently developed front end to the CONTAM simulation engine, ContamX.
Air Quality Testing: Methods and Techniques
You may want to see also
Simple Interactive Models for better air quality
Air pollution is a pressing issue for cities worldwide, especially in Asia, Latin America, and Africa. To address this challenge, scientists and policymakers have developed the Simple Interactive Models for better air quality (SIM-air) toolkit. This family of tools aims to utilise the best available information on all known sources of air pollution to support integrated air quality management.
The SIM-air toolkit offers a range of user-friendly features and resources. It includes an Air Quality Index (AQI) Calculator, which uses monitoring data to provide real-time or forecasted AQI estimates. Additionally, the Atmospheric Transport Modelling System (ATMoS) Dispersion Model generates transfer matrices for multiple sources and pollutant types. For vehicle-specific emissions, SIM-air provides V-Dust, a calculator for estimating fugitive dust emissions from vehicles.
One of the key strengths of SIM-air is its ability to simulate interactions between emissions, pollution dispersion, impacts, and management options. It achieves this through modules that estimate emissions and their subsequent interactions. These modules are maintained in spreadsheets for easy access and analysis. The toolkit also offers individual calculators for emissions and pollution management, allowing users to estimate parameters such as emissions from various sources.
The SIM-air toolkit has been applied in various contexts, including the Air Pollution Knowledge Assessments (APnA) city program. It was first introduced in 2000 for sulfur pollution management in power plants, and an integrated version was released in 2002 for PM2.5 pollution analysis. The current version of SIM-air is more robust, offering optimisation capabilities and compatibility with MS Excel for larger domains, multiple sectors, and multiple pollutants. Users can access manuals, primers, and a series of videos that provide instructions and insights into the components of the SIM-air toolkit.
Nitrogen's Air Pollution Impact: What You Need to Know
You may want to see also
Air pollution models for regulatory or policy assessments
Air pollution modelling is a method for providing information on air quality based on emissions and the atmospheric processes that lead to pollutant dispersion, transport, chemical conversion, and removal from the atmosphere by deposition. Air pollution models are a primary tool for analysis in most air quality assessments. They are used to predict future pollutant concentrations from multiple sources and assist in the design of strategies to reduce harmful air pollutants.
There are several types of air pollution models used for regulatory or policy assessments. Dispersion modelling, for example, is used in the permitting process to estimate the concentration of pollutants at specified ground-level receptors surrounding an emissions source. Photochemical modelling is used in regulatory or policy assessments to simulate the impacts from all sources by estimating pollutant concentrations and deposition over large spatial scales. Receptor modelling, on the other hand, is an observational technique that uses the chemical and physical characteristics of gases and particles to identify and quantify source contributions to receptor concentrations.
The US Environmental Protection Agency (EPA) also employs wind tunnel labs to study air flow and dispersion. These labs use scaled models to simulate the spread of harmful airborne material released in urban and industrial settings. The EPA's Fluid Modelling Facility Meteorological Wind Tunnel lab, for instance, uses a 1:200 scale model to study the impact of building shapes and sizes, as well as their orientation to streets, on the quantity and direction of pollutants in the wind flow. This data is then used to inform decision-making on air quality issues and evaluate mitigation options.
In addition to these methods, the European Environment Agency (EEA) also utilizes air pollution modelling to assess urban air quality. Their models take into account various source categories, such as line, point, and area sources, and consider the largest point sources individually in the calculation. For reactive pollutants, chemical modules are included in the model to describe photochemical reactions, such as the transformation of SO2 into sulfates or the formation of ozone and NOX.
Furthermore, the SIM-air family of tools was developed to utilize the best available information on all known sources of air pollution to support integrated air quality management. These tools are designed to estimate emissions, simulate interactions between emissions, pollution dispersion, impacts, and management options, and optimize management strategies for cost, health impacts, or compliance with standards.
Understanding SO3: Air Pollutant Criteria and Implications
You may want to see also
Frequently asked questions
The SIM-air family of tools was developed to use the best available information on all the known sources of air pollution to support integrated air quality management. The modules are designed to estimate emissions and simulate the interactions between emissions, pollution dispersion, impacts, and management options.
In a wind tunnel, researchers can control everything, including wind direction, source locations, and the amount of pollutant. The models used in the wind tunnel are usually designed and handmade by the researchers, using materials like wood or foam.
The IAQX simulation tool kit is an indoor air quality (IAQ) simulation software package that complements and supplements existing indoor air quality simulation (IAQ) programs. IAQX is for advanced users who have experience with exposure estimation, pollution control, risk assessment, and risk management.
Some of the most commonly used air quality models include dispersion modeling, photochemical modeling, and receptor modeling. Dispersion models estimate the concentration of pollutants at ground-level receptors surrounding an emissions source, while photochemical models simulate the impacts from all sources by estimating pollutant concentrations over large spatial scales. Receptor models use observational techniques to identify and quantify source contributions to receptor concentrations.
Air pollution simulation tools have a variety of applications, including urban planning, policy assessments, and risk management. For example, air quality models can be used during the permitting process to verify that a new source of emissions will not exceed ambient air quality standards.