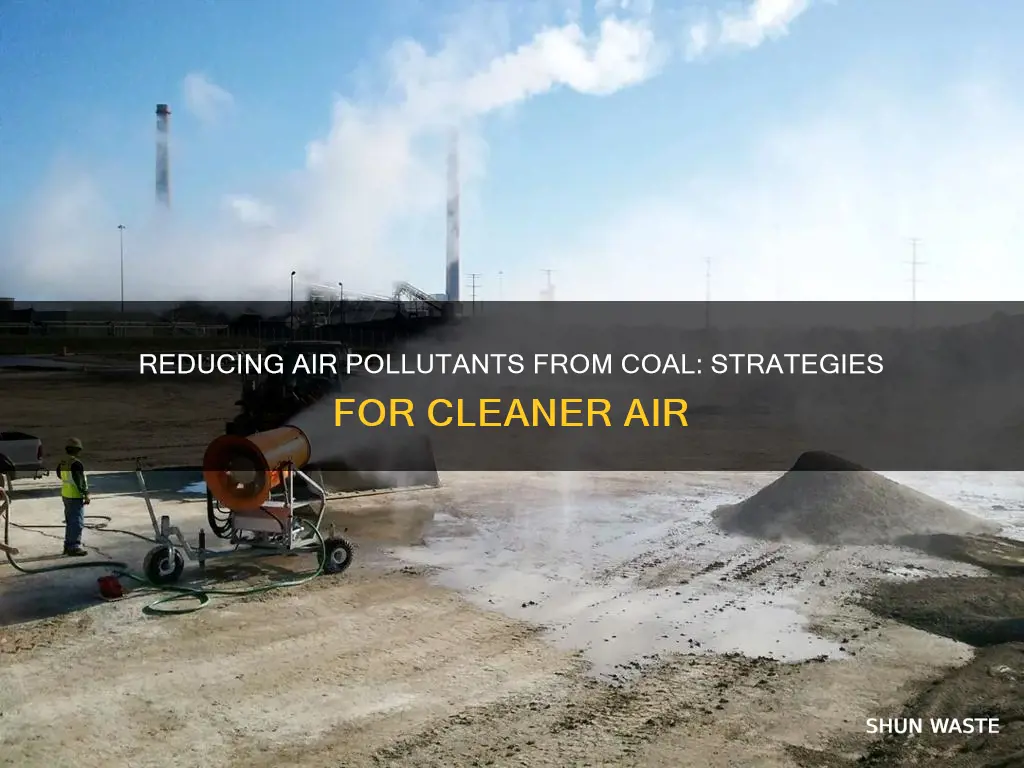
Burning coal releases harmful substances that contribute to air pollution, acid rain, and greenhouse gas emissions. Mitigation measures include pre-combustion approaches, such as cleaning coal, and post-combustion approaches, including flue-gas desulfurization, selective catalytic reduction, electrostatic precipitators, and fly ash reduction. These measures aim to reduce the impact of coal on human health and the environment.
Coal-fired power plants emit toxins and pollutants such as mercury, lead, sulfur dioxide, nitrogen oxides, particulates, and various other heavy metals. These emissions can cause asthma, breathing difficulties, brain damage, heart problems, cancer, neurological disorders, and premature death.
The combustion of coal also produces waste in the form of coal ash, which often contains arsenic, mercury, and lead, and can contaminate waterways and drinking water supplies if not properly disposed of.
Several methods can be used to reduce air pollutants from coal:
- Flue-gas desulfurization: This technology is used to remove sulfur dioxide (SO2) from the flue gas before it exits the smokestack.
- Selective catalytic reduction (SCR): This technology reduces nitrogen oxides (NOx) emissions.
- Electrostatic precipitators: These devices remove particulate matter from the smoke.
- Carbon capture and storage (CCS): This technology separates and captures CO2 from emissions sources, which can then be stored underground.
- Oxy-fuel combustion: This method burns coal in a mixture of recirculated flue gas and oxygen, reducing the volume of exhausted flue gas and nitrogen emissions.
- Activated carbon injection (ACI): This method removes mercury emissions and is the preferred technique in many countries.
- Advanced dry scrubbers: These scrubbers can be used in conjunction with circulating fluidized beds to increase the removal of mercury.
- Wet scrubbers: These can be integrated with existing wet scrubbers or retrofitted to dry electrostatic precipitators to reduce particulate matter emissions.
- Energy-efficient processes: Improving energy efficiency in coal-fired power plants can reduce the amount of coal burned and lower emissions.
- Switch to carbon-neutral fuels: Transitioning to renewable energy sources, such as hydro, solar, and wind power, can help reduce greenhouse gas emissions and improve air quality.
Characteristics | Values |
---|---|
Cleaning coal | Physical and chemical treatments |
Flue-gas desulfurization | Removes SO2 |
Selective catalytic reduction | Removes NO2 |
Electrostatic precipitators | Remove particulates |
Fly ash reduction | Reduces emissions of radioactive materials |
Carbon capture | Pre-combustion, post-combustion, oxy-fuel combustion |
What You'll Learn
- Use flue-gas desulfurization to remove sulfur dioxide
- Use selective catalytic reduction to remove nitrogen oxides
- Use electrostatic precipitators to remove particulates and heavy metals
- Reduce fly ash to limit emissions of radioactive materials
- Use carbon capture and storage technologies to reduce carbon dioxide emissions
Use flue-gas desulfurization to remove sulfur dioxide
Flue-gas desulfurization (FGD) is a set of technologies used to remove sulfur dioxide (SO2) from the exhaust flue gases of fossil-fuel power plants, as well as from the emissions of other sulfur oxide-emitting processes such as waste incineration, petroleum refineries, and cement and lime kilns.
As an acidic gas, SO2 needs to be neutralised before disposal. This is done using an alkaline-based sorbent, which brings the pH level of the sulfur dioxide gas closer to neutral.
FGD systems can remove 90% or more of the SO2 in flue gases. There are two main types of FGD: wet scrubbing and dry scrubbing.
Wet Scrubbing
Wet scrubbing uses a slurry of alkaline sorbents, usually limestone or lime, or seawater to scrub gases. The flue gas exits the water tube boiler and is drawn into the scrubber spray tower by a fan. The flue gas will usually be in excess of 150 degrees Celsius, so it may be passed through a heat exchanger before entering the spray tower. The lower third of the spray tower is where the alkaline-based slurry is held, known as the effluent holding tank (EHT). The slurry is most commonly a mixture of ground-up limestone and water. A heavy-duty centrifugal pump is used to pull the slurry through and discharge it from the spray headers nearer the top of the tower. The slurry gets sprayed across the entire tray area where the gas meets the slurry. As the sulfur dioxide comes into contact with the slurry, it is absorbed by the water and neutralised by the limestone within the slurry. The gas that continues to rise is now at a pH level closer to neutral, and the slurry then drops back down into the EHT. Any remaining slurry that continues to rise is stopped by a mist eliminator. The sulfur dioxide gases are now neutralised, and the reaction that has taken place in the slurry has produced calcium sulphite (CaSO3) which, in turn, can be turned into calcium sulphate (CaSO4) using a process known as forced oxidation.
Oxygen in the form of compressed air is drawn directly into the slurry in the EHT via nozzles from a compressed airline. This bubbles air into the slurry, enabling the calcium sulphite to oxidise into calcium sulphate, also known as gypsum. This step is advantageous as gypsum can be sold as a byproduct, helping with cost-effectiveness, and it is also easier to separate from the water than calcium sulphite. The calcium sulphite will gradually settle out to the base of the tank where it can be extracted via a discharge hole. Around 15% of the discharged slurry will contain the suspended solids, including gypsum, now referred to as sludge. Once the gypsum is discharged, it is sent for dewatering, where it can be used as a byproduct for the cement and agricultural industries.
Dry Scrubbing
Dry scrubbing uses dry sorbents, such as powdered hydrated lime, injected into the exhaust ducts to eliminate SO3 from process emissions.
Reducing Urban Noise Pollution: Strategies for Quieter Cities
You may want to see also
Use selective catalytic reduction to remove nitrogen oxides
Nitrogen oxides (NOx) are one of the most significant air pollutants, causing smog, respiratory illnesses, and acid rain. They are produced during the combustion of fossil fuels, such as coal, and can lead to serious damage to the environment and human health. To remove NOx from coal emissions, selective catalytic reduction (SCR) is a widely used and effective method.
SCR technology involves injecting ammonia into the exhaust stream, which then reacts with NOx in the presence of a catalyst. This reaction converts NOx into harmless water vapour and nitrogen gas, reducing air pollution and its associated health risks. The efficiency of SCR systems has been tested on diesel engines, showing a 95% reduction in NOx emissions.
While SCR is a valuable technique, it is important to note that the process requires the addition of ammonia, which can be expensive and may have its own environmental impacts. Additionally, the catalyst used in SCR systems must be carefully selected to ensure high activity and efficiency. Overall, SCR is a promising method for removing NOx from coal emissions, but further research and development are needed to optimise the process and minimise any potential drawbacks.
Recycling: Reducing Ocean Pollution, Saving Marine Life
You may want to see also
Use electrostatic precipitators to remove particulates and heavy metals
Electrostatic precipitators are large industrial emission control units used to remove dust from exhausted gases. They are highly effective at reducing particle pollution, including harmful particulate matter from waste gases at industrial facilities and power-generating stations. Electrostatic precipitators can capture fine particles, even those smaller than 2.5 microns in diameter, by charging and then moving them to collecting surfaces through the application of high-voltage direct current.
The basic construction of an electrostatic precipitator consists of a row of thin vertical rods and a stack of flat parallel metal plates. The contaminated gas flows through the space between the rods and then between the plates. A large negative voltage of 40-100 kV is applied between the rods and the metal plates to ionize the gas as it passes through. The ionized particles are attracted to the grounded metal plates, removing them from the gas stream. The particles then accumulate on the collection plates and are removed through mechanical rappers, falling into a hopper at the unit's bottom.
Electrostatic precipitators are often used in conjunction with denitrification units and scrubbers to remove nitrogen oxides and sulfur dioxide, respectively. They can handle large volumes of gas at various temperatures and flow rates and are available in many sizes and types, designed for different dust and water droplet characteristics. They are highly efficient in their consumption of energy, as they apply energy only to the particulate matter being collected.
The performance of electrostatic precipitators is influenced by factors such as collection surface area, gas-flow rate, gas temperature, and particle properties such as size distribution and electrical resistivity. Particles with high resistivity are challenging to charge and release their acquired charge slowly, while those with low resistivity are easily charged and quickly lose their charge. ESPs work optimally with particles of normal resistivity, which neither rapidly release nor retain their charge.
Strategies for Factories to Cut Pollution and Improve Sustainability
You may want to see also
Reduce fly ash to limit emissions of radioactive materials
Fly ash is a residue created when coal is burned for energy. It is one of the largest quantities of waste disposed of worldwide. The use of fly ash depends on its chemical, mineralogical composition, and morphology. The main amount of fly ash is used as an additive in concrete building products.
The concentration of radioactive elements in fly ash depends on the concentration of radioactive elements in the coal that is burned. The radioactivity of fly ash is not significantly different from that of conventional concrete additives or other building materials. However, fly ash can contain elevated concentrations of radioactive materials compared to the parent coal. The radioactivity of coals and coal combustion products has raised worldwide attention since the 1960s.
The partitioning of radioactive elements between gas and solid waste is controlled by volatility and chemistry. Almost 100% of the radon gas present in feed coal is transferred to the gas phase and lost in stack emissions. In contrast, less volatile elements such as thorium, uranium, and the majority of their decay products are retained in the solid combustion wastes. The average ash yield of coal burned in the United States is approximately 10 weight percent. Therefore, the concentration of most radioactive elements in solid combustion wastes will be approximately 10 times the concentration in the original coal.
The uranium concentration of most fly ash (10 to 30 ppm) is still in the range found in some granitic rocks, phosphate rocks, and shales. For example, the Chattanooga Shale, which occurs in a large portion of the Southeastern United States, contains between 10 and 85 ppm of uranium. Most thorium in coal is contained in common phosphate minerals such as monazite or apatite. In contrast, uranium is found in both the mineral and organic fractions of coal.
The use of materials containing natural radioactive nuclides in the construction industry is controlled by legal agencies to examine the radioactive dose levels. Acceptable radioactivity levels are a key factor for the safe utilization of wastes and by-products of coal-burning power plants. In general, the radioactivity levels of most fly ashes are similar to natural materials. For higher radioactivity fly ash, the radioactivity values must be reduced to acceptable limits. This can be done by mixing the fly ash with less radioactive natural materials.
One technique to reduce the radioactivity of fly ash involves the use of snow as an additive to the compaction water of fly ash. The fly ash is compacted at optimum water content, and fly ash with additional 10% by weight snow is compacted, hermetically sealed to allow for equilibrium of 226Ra and 232Th with their decay products, and cured for 28 days. The activities of 235U, 226Ra, 238U, and 232Th of the fly ash and snow-added fly ash samples compacted at optimum moisture content are determined. The control samples revealed radioactivity values above the maximum allowable limits. The addition of snow caused a decrease of 31–42% in the radioisotope activity levels compared to the control samples.
The decrease in radioactivity is linked to an increased void ratio after the melting of ice and increased densification of the matrix around the pores due to a higher level of cementitious mineral formation. The decrease in the radioisotope activity levels will allow the utilization of fly ash in highway embankment construction, where large surface area exposure and large volume usage make it more critical for human health.
Ways to Reduce Water Pollution and Save Our Oceans
You may want to see also
Use carbon capture and storage technologies to reduce carbon dioxide emissions
Carbon capture and storage (CCS) technologies are an effective way to reduce carbon dioxide emissions from coal. CCS is a three-step process that involves capturing, transporting, and storing carbon dioxide produced during industrial processes or the burning of fossil fuels.
The first step, capturing the CO2, involves separating it from other gases produced during industrial processes or fossil fuel combustion. This can be done through post-combustion capture, pre-combustion capture, or oxy-combustion. Post-combustion capture removes CO2 after fossil fuel combustion, while pre-combustion capture involves partially oxidizing the fossil fuel to produce syngas, which is then used to generate power. Oxy-combustion, on the other hand, involves burning fuel in pure oxygen instead of air, resulting in a flue gas consisting mostly of CO2 and water vapour.
Once captured, the CO2 is compressed and transported via pipelines, ships, or other means to a storage site. Finally, the CO2 is injected into deep underground geological formations, such as depleted oil and gas reservoirs or saline aquifers, for permanent storage.
CCS can play a crucial role in tackling global warming and reducing carbon emissions. It is especially useful for heavy industries like cement, steel, and chemicals, which are hard to abate through other means. Additionally, CCS can be retrofitted to existing power and industrial plants, enabling their continued operation while reducing emissions.
While CCS has the potential to mitigate climate change, it also faces challenges and limitations. CCS facilities require more energy to operate, leading to increased fuel consumption and pollution. It is also expensive compared to other emission reduction methods like solar, wind energy, and electrification.
CCS has been in operation since 1972, with natural gas plants in Texas capturing and storing large amounts of CO2 underground. As of 2024, CCS was in operation at 44 plants worldwide, capturing about one-thousandth of greenhouse gas emissions. The effectiveness of CCS depends on factors such as capture efficiency, additional energy usage, leakage, and technical issues.
Overall, CCS is a promising technology for reducing carbon dioxide emissions, but it needs to be complemented by a broader shift to renewable energy and other emission reduction strategies.
How Paper Recycling Helps Fight Pollution
You may want to see also
Frequently asked questions
When coal is burned, it releases a number of airborne toxins and pollutants, including mercury, lead, sulfur dioxide, nitrogen oxides, particulates, and various other heavy metals.
Health impacts can range from asthma and breathing difficulties to brain damage, heart problems, cancer, neurological disorders, and premature death.
Coal pollution can be reduced by cleaning coal before it is burned, and by using flue-gas desulfurization, selective catalytic reduction, electrostatic precipitators, and fly ash reduction after it is burned.