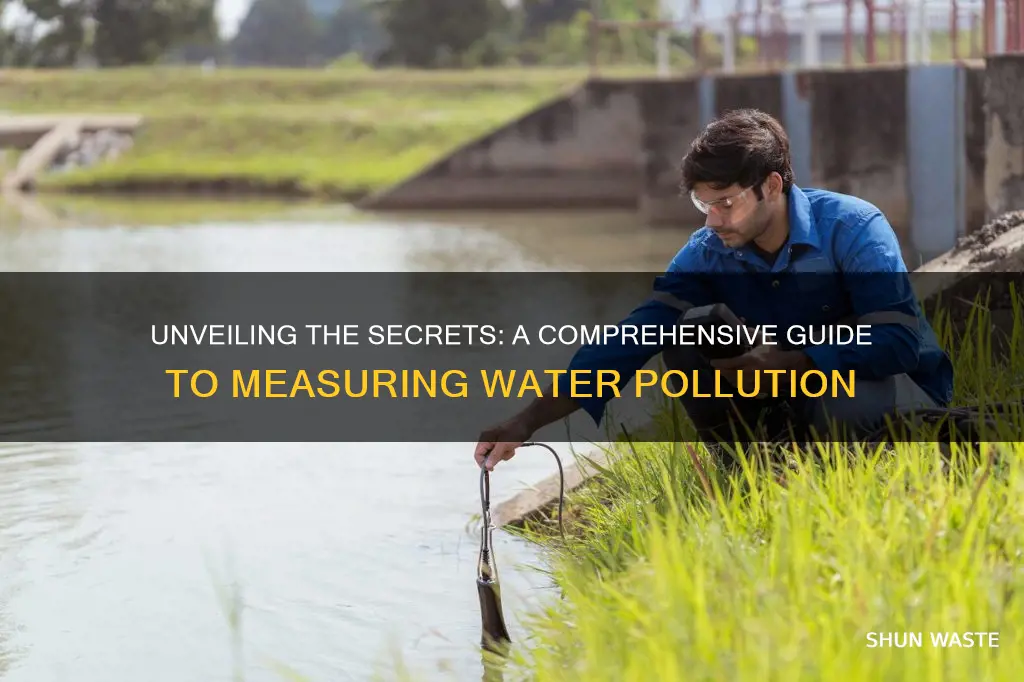
Water pollution is a critical environmental issue, and accurately measuring its extent is essential for effective management and conservation efforts. This paragraph introduces the topic of assessing water pollution levels, highlighting the importance of various methods and tools used to quantify and monitor the quality of water bodies. It emphasizes the need for comprehensive approaches that consider both physical and chemical parameters to ensure a thorough understanding of pollution levels and their sources.
What You'll Learn
- Water Quality Parameters: Measure pH, dissolved oxygen, turbidity, and temperature
- Biological Indicators: Use bacteria, algae, and fish populations as pollution sensors
- Chemical Analysis: Test for heavy metals, nutrients, and toxic substances
- Remote Sensing: Satellite data can detect water quality changes over large areas
- Citizen Science: Engage local communities in monitoring water quality
Water Quality Parameters: Measure pH, dissolved oxygen, turbidity, and temperature
Water quality is a critical aspect of environmental health, and assessing various parameters is essential to understanding the state of our water bodies. Here, we delve into four key indicators: pH, dissolved oxygen, turbidity, and temperature, each playing a significant role in gauging water pollution levels.
PH: This parameter measures the acidity or alkalinity of water, indicated on a scale from 0 to 14. A pH of 7 is neutral, with values below 7 being acidic and above 7 alkaline. Water pollution often disrupts this balance. For instance, acidic water, with a pH below 6, can result from industrial waste or acid rain, posing risks to aquatic life. Conversely, alkaline water (pH above 8) may indicate the presence of certain pollutants or natural mineral deposits. Regular pH testing is crucial for identifying these issues and ensuring water safety.
Dissolved Oxygen (DO): DO is a vital parameter, as it directly affects aquatic organisms' survival. It is measured in milligrams per liter (mg/L) or parts per million (ppm). Healthy water typically has DO levels above 8 mg/L. Low DO levels, often below 4 mg/L, can be detrimental, leading to fish kills and other ecological imbalances. Common causes of DO depletion include organic pollution from agricultural runoff or sewage, which introduces excess nutrients and organic matter into water bodies. Monitoring DO is essential for maintaining aquatic ecosystems and preventing water quality degradation.
Turbidity: This measure assesses the cloudiness or clarity of water, providing insights into its transparency. Turbidity is expressed in forms such as Formazin turbidity units (FTU) or nephelometric turbidity units (NTU). High turbidity, often exceeding 50 NTU, can obscure the water's natural color and reduce light penetration, impacting aquatic plants and animals. Sources of turbidity include sediment runoff from construction sites, erosion, and organic matter decomposition. Regular turbidity testing is vital for water quality management, especially in recreational waters and drinking water sources.
Temperature: Water temperature is a critical factor influencing aquatic life and pollution levels. It is measured in degrees Celsius or Fahrenheit. Warmer water temperatures can accelerate the growth of harmful algae blooms and increase the solubility of certain pollutants. Conversely, colder temperatures may limit the activity of certain microorganisms responsible for breaking down pollutants. Temperature monitoring is essential for understanding water body dynamics and predicting pollution impacts.
In summary, these four parameters—pH, dissolved oxygen, turbidity, and temperature—are fundamental tools for assessing water quality and pollution levels. Each indicator provides unique insights, allowing for timely interventions and effective water management strategies. Regular monitoring and analysis of these parameters are essential for preserving the health of our water resources and the ecosystems they support.
Water's Conductivity: A Key Indicator of Pollution's Reach
You may want to see also
Biological Indicators: Use bacteria, algae, and fish populations as pollution sensors
Water pollution is a critical environmental issue, and assessing its severity is essential for implementing effective mitigation strategies. Biological indicators, which rely on the sensitivity of aquatic organisms to pollutants, offer a unique and natural approach to monitoring water quality. This method involves studying the presence and health of bacteria, algae, and fish populations as indicators of pollution levels.
Bacteria are often the first responders to water pollution. Certain bacterial species can rapidly detect changes in water quality. For instance, *Pseudomonas* and *Escherichia coli* are commonly used as bioindicators. These bacteria can survive and thrive in various environments, but their growth rates and survival patterns can be significantly influenced by pollutants. When pollutants are introduced into a water body, these bacteria may exhibit altered metabolic activities, such as increased respiration rates or changes in cell structure, providing valuable insights into the presence and concentration of contaminants.
Algae, particularly phytoplankton, also play a crucial role in biological pollution monitoring. Algal blooms, which are rapid increases in algal populations, can be indicative of nutrient pollution, often caused by excessive nutrient runoff from agricultural activities or urban areas. Excessive nutrients, such as nitrogen and phosphorus, stimulate algal growth, leading to dense algal populations. Scientists can monitor these blooms to assess the impact of pollution on water ecosystems. Additionally, the composition and diversity of algal species present in a water sample can provide information about the overall health of the aquatic environment.
Fish populations are another vital component of biological indicators. Fish are highly sensitive to changes in water quality, and their presence or absence, as well as the diversity and abundance of species, can provide valuable pollution-related data. For example, the decline or disappearance of certain fish species in a water body may indicate severe pollution, as these species often have specific habitat requirements and are sensitive to even minor changes in water chemistry. By studying fish populations, scientists can identify the specific pollutants affecting a water body and assess the overall ecological health.
In summary, biological indicators, including bacteria, algae, and fish populations, offer a natural and effective means of measuring water pollution levels. These organisms respond to pollutants in unique ways, allowing scientists to gather valuable data on water quality. By monitoring bacterial metabolic activities, algal blooms, and fish populations, researchers can identify pollution sources, assess pollution severity, and develop strategies to protect and restore aquatic ecosystems. This approach provides a sustainable and environmentally friendly method for long-term water quality monitoring and management.
Septic Tanks: Nature's Filter for Clean Water
You may want to see also
Chemical Analysis: Test for heavy metals, nutrients, and toxic substances
Chemical analysis plays a crucial role in assessing water pollution levels, especially when it comes to identifying and quantifying various contaminants. One of the primary methods within this field is testing for heavy metals, nutrients, and toxic substances, which can provide valuable insights into the health of aquatic ecosystems.
Heavy metals, such as lead, mercury, cadmium, and arsenic, are of significant concern due to their persistence in the environment and potential toxicity. These metals can enter water bodies through industrial discharge, agricultural runoff, or natural geological processes. To measure their concentration, specialized techniques are employed. Atomic absorption spectroscopy (AAS) and inductively coupled plasma mass spectrometry (ICP-MS) are commonly used instruments. These methods allow for the detection and quantification of trace amounts of heavy metals, ensuring that even low levels of contamination are identified. For instance, AAS measures the absorption of light by ground-state atoms at specific wavelengths, while ICP-MS provides highly sensitive and precise measurements by ionizing the sample and detecting the mass-to-charge ratio of metal ions.
Nutrients, including nitrogen and phosphorus compounds, are essential for aquatic life, but excessive amounts can lead to eutrophication, causing harmful algal blooms and oxygen depletion. Chemical analysis techniques like inductively coupled plasma optical emission spectroscopy (ICP-OES) and colorimetry are utilized to determine nutrient levels. ICP-OES offers high sensitivity and selectivity, enabling the detection of multiple nutrients simultaneously. It measures the intensity of light emitted at specific wavelengths, providing accurate concentrations. Colorimetry, on the other hand, relies on the color change of a solution when it reacts with a nutrient, offering a simpler and more cost-effective approach for routine monitoring.
Toxic substances, such as pesticides, pharmaceuticals, and industrial chemicals, require specialized testing methods due to their diverse chemical structures and potential environmental impacts. Liquid chromatography-tandem mass spectrometry (LC-MS/MS) is a powerful technique for identifying and quantifying these compounds. It involves separating and analyzing the components of a complex mixture, allowing for the detection of even trace amounts of toxic substances. This method is highly sensitive and can provide detailed information about the chemical composition of water samples, aiding in the identification of emerging contaminants.
In summary, chemical analysis is a vital tool for assessing water pollution, particularly when targeting heavy metals, nutrients, and toxic substances. Each of these contaminants requires specific testing methods to ensure accurate measurements. By employing techniques like AAS, ICP-MS, ICP-OES, colorimetry, and LC-MS/MS, scientists and environmental agencies can gather comprehensive data to monitor and manage water quality effectively, contributing to the preservation of aquatic ecosystems and public health.
Water's Dark Turn: Unveiling the Impact of Pollution
You may want to see also
Remote Sensing: Satellite data can detect water quality changes over large areas
Remote sensing technology, particularly satellite imagery, has revolutionized the way we monitor and assess water quality over vast regions. This method allows scientists and environmental agencies to gather valuable data without physically collecting samples, making it an efficient and cost-effective tool for water pollution monitoring. The process involves using various sensors and algorithms to detect and analyze specific parameters in water bodies, such as lakes, rivers, and coastal areas.
Satellite data can capture a wide range of water quality indicators. For instance, it can measure the concentration of chlorophyll, a pigment found in algae, which indicates the presence of phytoplankton. High levels of chlorophyll can suggest nutrient pollution, often caused by agricultural runoff or sewage discharge, leading to harmful algal blooms. These blooms can deplete oxygen levels in the water, creating 'dead zones' where aquatic life cannot survive. By monitoring chlorophyll levels, satellites can help identify areas at risk of such ecological disasters.
Another critical parameter that satellites can detect is water turbidity, which is the cloudiness or opacity of water caused by suspended particles. Turbidity can be a result of sediment runoff, industrial waste, or natural processes. High turbidity levels can affect water quality, making it unsuitable for drinking or recreational use. Satellite sensors can measure turbidity by analyzing the light reflected or scattered by the water, providing a non-invasive way to monitor this essential water quality parameter.
Furthermore, remote sensing can identify changes in water temperature, which is a significant factor in aquatic ecosystem health. Warmer water temperatures can lead to reduced oxygen levels and the migration of fish species, impacting the overall biodiversity of an area. Satellites equipped with thermal sensors can detect these temperature variations, allowing scientists to study the thermal dynamics of water bodies and their effects on pollution levels.
The power of remote sensing lies in its ability to provide a comprehensive view of water bodies, covering vast areas that would be challenging and time-consuming to survey using traditional methods. Satellite data can be collected regularly, ensuring a consistent and up-to-date record of water quality. This information is invaluable for water resource management, environmental policy-making, and the early detection of pollution events, enabling swift action to mitigate potential ecological and health risks.
Unveiling the Hidden Dangers: Common Ways to Pollute Water
You may want to see also
Citizen Science: Engage local communities in monitoring water quality
Citizen science initiatives can play a vital role in monitoring and assessing water quality, especially in areas where traditional monitoring methods may be limited or costly. Engaging local communities in water quality monitoring empowers citizens to take an active role in protecting their environment and can provide valuable data for scientists and policymakers. Here's how this approach can be implemented:
Training and Education: Begin by educating community members about water pollution, its sources, and potential impacts. Provide practical training on water testing techniques, including the use of simple, portable testing kits. These kits can measure various parameters such as pH, dissolved oxygen, turbidity, and the presence of contaminants like heavy metals or pesticides. Ensure that participants understand the importance of accurate data collection and the potential health and environmental risks associated with polluted water.
Establish Citizen Science Networks: Create a network of volunteers within the community who are passionate about water conservation. These volunteers can form local water monitoring teams, each equipped with the necessary testing tools and knowledge. Regular meetings or workshops can be organized to share best practices, discuss findings, and provide updates on water quality trends in the area. This collective effort fosters a sense of ownership and encourages long-term commitment to the project.
Data Collection and Analysis: Local teams can collect water samples from various sources, including rivers, lakes, and coastal areas, depending on the specific water bodies of concern. They should record detailed information about the sampling location, date, and time. By following standardized testing protocols, volunteers can measure water quality parameters and record the results. This data can then be compiled and analyzed to identify patterns, sources of pollution, and potential hotspots. Advanced data analysis techniques, such as statistical modeling, can be employed to interpret the findings.
Community Engagement and Communication: Effective communication is key to the success of citizen science projects. Share the results of water quality monitoring with the local community through reports, newsletters, or public meetings. Visual representations, such as maps or graphs, can help illustrate the data and engage a wider audience. By making the information accessible and understandable, citizens can better grasp the state of their local water bodies and the actions needed to improve them. This transparency can also foster trust and encourage community members to take further initiatives to protect their water resources.
Long-term Monitoring and Advocacy: Citizen science projects should aim for sustainability and continuity. Regular monitoring over an extended period allows for the tracking of water quality changes and the identification of long-term trends. This data can be crucial in advocating for policy changes, raising awareness, and holding stakeholders accountable for water pollution prevention and control. Local communities can also use this information to propose solutions, such as community-led clean-up initiatives or advocacy for stricter regulations on industrial waste discharge.
Clean Water Fun: Simple Steps for Kids to Purify Water
You may want to see also
Frequently asked questions
Water pollution levels can be assessed through various methods, including water sampling and analysis. Common techniques involve measuring chemical concentrations, such as dissolved oxygen, pH, nutrient levels (nitrates, phosphates), and the presence of pollutants like heavy metals, pesticides, and organic compounds. These measurements provide insights into the water's quality and potential sources of contamination.
Scientists often use bioassays and biomonitoring to assess the ecological impact of pollution. This involves studying the effects of contaminated water on aquatic organisms, such as fish, algae, or bacteria. By observing changes in growth, reproduction, or behavior, researchers can determine the level of pollution and its potential harm to the ecosystem.
Yes, several field tests can provide quick estimates of water pollution. For instance, the water's color and clarity can indicate the presence of suspended solids or organic matter. Simple tests for pH, using indicators or pH meters, can give an idea of the water's acidity. Additionally, measuring dissolved oxygen levels with a probe can help assess the water's oxygen availability, which is crucial for aquatic life.
Remote sensing technologies, such as satellite imagery and aerial surveys, are valuable tools for monitoring water bodies over large areas. These methods can detect changes in water color, identify potential pollution sources like industrial discharge or agricultural runoff, and provide data on water quality. Remote sensing data is essential for mapping pollution hotspots and supporting environmental management decisions.