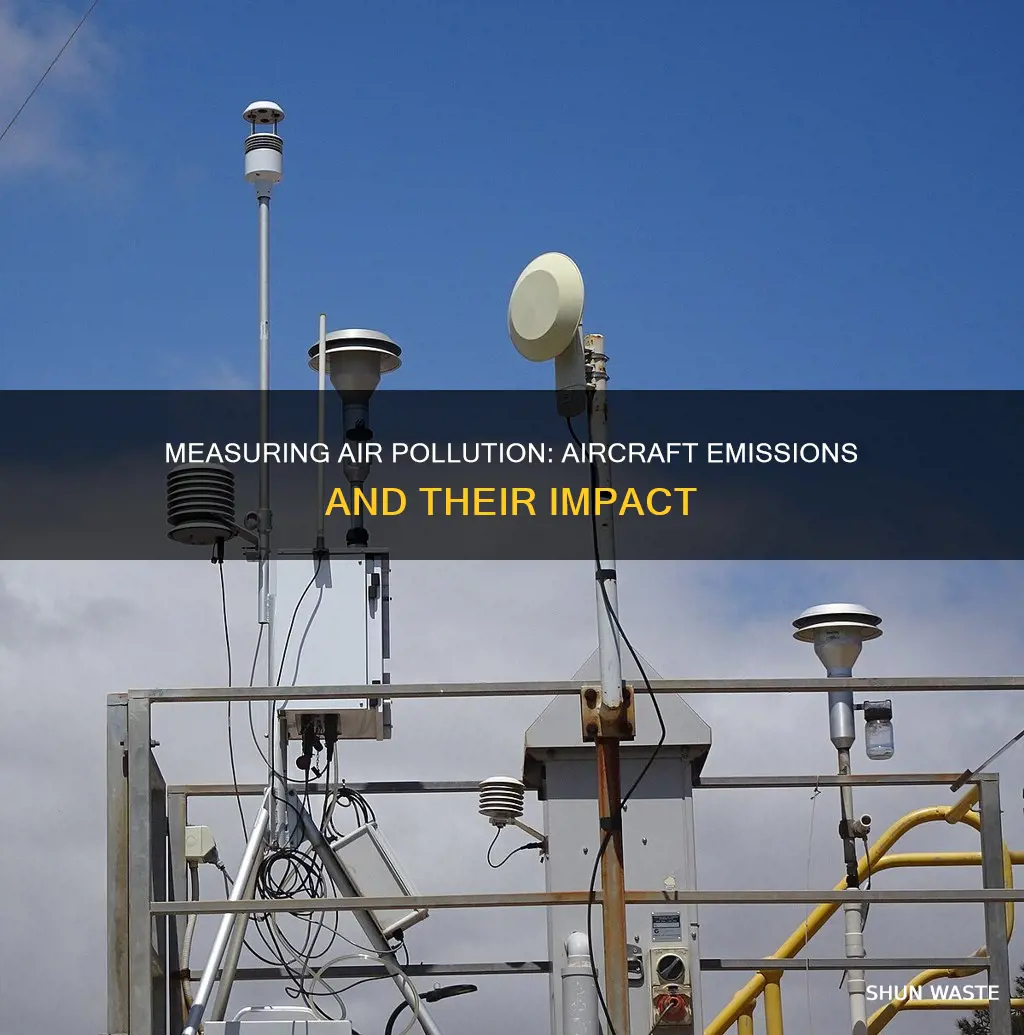
Air pollution caused by airplanes has been a growing concern for environmentalists and governments alike. With the world witnessing an increase in air traffic, the need to measure and control air pollution caused by airplanes has become more crucial than ever. Various studies have been conducted to assess the impact of aviation emissions on air quality, with findings suggesting that it leads to approximately 58,000 premature deaths annually. To address this issue, organizations like the EPA have proposed and implemented emission standards for aircraft engines, aiming to reduce their carbon footprint and improve air quality. Additionally, smart technologies and innovative measurement techniques, such as MOZAIC, are being employed to monitor and mitigate the effects of aviation on the atmosphere. As the awareness of aviation's impact on air quality grows, further research and stricter regulations are expected to be implemented to ensure a sustainable future for the aviation industry.
Characteristics | Values |
---|---|
Air temperature | Measured in the supply air as it enters a zone, or in the cabin or cockpit air after the supplied air mixes with the resident air |
Barometric pressure | Continuously measured and under the precise control of an automatic system |
Carbon monoxide | Peak concentrations of about 5 ppm |
RSP | Peak concentrations of about 500 μg/m3 |
Ozone | Mean O3 concentration in the cabin exceeded 0.20 ppm |
Emission standards | The EPA proposed GHG emission standards for engines of certain new commercial airplanes, including all large passenger jets |
Aviation emissions | Resulted in 21,200 premature mortalities due to particulate matter exposure and 53,100 due to ozone exposure |
What You'll Learn
Measuring carbon monoxide and RSP
Carbon monoxide (CO) is a colorless, odorless, and toxic gas that can quickly reach deadly levels in enclosed spaces. To prevent carbon monoxide poisoning, various instruments and devices are used to measure its concentration. Real-time measuring devices are available at moderate prices, and they can be used to monitor CO levels continuously. Federal agencies like the EPA (Environmental Protection Agency) and OSHA (Occupational Safety and Health Administration) play a crucial role in setting standards and guidelines to ensure that CO levels are maintained at safe levels. These agencies also work to regulate and reduce greenhouse gas emissions from aircraft, as required by the Clean Air Act.
RSP, or respirable suspended particles, are also of significant concern in airplane cabins. RSP can include fine particulate matter, such as smoke, dust, or aerosols, which can have adverse health effects when inhaled. To measure RSP concentrations, instruments like hand-held nephelometers and piezoelectric balances have been used. These devices measure the mass concentration of suspended particulate matter, with nephelometers being particularly effective for particles in the submicrometer range. Federal standards for indoor air quality, such as the Japanese standard of 150 μg/m3 for particle density, provide benchmarks for acceptable RSP levels.
The measurement of carbon monoxide and RSP is often used as surrogates to detect ETS (environmental tobacco smoke). ETS is a complex mixture of many components, and the concentration of ETS is influenced by factors such as the number of active smokers, ventilation rates, and air recirculation systems. By measuring carbon monoxide and RSP levels, we can gain insights into the overall air quality and take appropriate actions to mitigate the impact of air pollution from airplanes.
It is important to note that data on carbon monoxide and RSP concentrations in airplane cabins may be limited and require further peer review. Additionally, standards for particle mass, such as the TSP (Total Suspended Particles) standard, may not adequately address the toxicity or size distribution of RSP, which can have unique health implications. Therefore, the development of specific standards for RSP and continued research into accurate measurement techniques are crucial for effectively managing air pollution from airplanes.
Human Actions to Reduce Air Pollution
You may want to see also
Monitoring O3 and water vapour
To address this, a sampling program called Measurement of Ozone and Water Vapour by Airbus In-Service Aircraft (MOZAIC) was launched in 1983 by European scientists. MOZAIC aims to measure O3 and water vapour in the atmosphere using commercial long-range aircraft. The program utilises fully automatic instruments installed on five long-range Airbus 340 aircraft from carriers such as Air France, Sabena, Lufthansa, and Austrian Airlines. By the end of December 1997, MOZAIC had completed 7,500 flights and recorded 54,000 flight hours of observation. While MOZAIC primarily focuses on O3 outside the aircraft, the same methodology could be applied to monitor O3 and other characteristics inside the aircraft.
The MOZAIC program employs a dual-beam ultraviolet-photometric instrument to measure O3 by monitoring its absorbance at 254 nm. This instrumentation stands out for its ruggedness, reliability, accuracy, and sensitivity (approximately 1 ppb with a 10-cm pathlength). Additionally, it consumes no consumables and maintains calibration effectively. The primary sampling point for measuring O3 is the air supplied to the passenger cabin, as it provides the best indication of O3 catalyst efficacy and occupant exposure.
Continuous O3 monitoring is crucial for evaluating the necessity of O3-removing devices on different flight routes. While O3-destroying catalysts are commonly used on high-altitude polar flights, data from NASA in the late 1970s revealed that high O3 levels can also occur on flights at lower latitudes, particularly during late winter and early spring. This highlights the importance of ongoing O3 monitoring to ensure the health and safety of passengers and crew.
Geo Power Plants: Air Pollution or Clean Energy?
You may want to see also
Assessing the impact of aircraft idling
Aircraft idling is a significant contributor to air pollution and has adverse health effects. To assess the impact of aircraft idling, various methods and models have been developed. One approach is to use detailed chemistry-transport models, such as the Community Multiscale Air Quality (CMAQ) model, which takes into account gas-phase chemistry, particulate matter, and air toxics. However, this method is computationally intensive and may not allow for the assessment of a wide range of policy options.
An alternative is to use surrogate modelling approaches, which enable a rapid assessment of the impact of aviation emissions scenarios on public health. For example, a study in 2005 used CMAQ simulations and an aircraft emissions inventory to estimate that 210 deaths per year were attributable to aircraft emissions, with a total monetized value of $1.4 billion per year in year 2000 US dollars. This type of modelling can also be applied to assess the health impacts of different environmental policies, such as low-sulfur fuel standards and NOx regulatory interventions.
Another way to measure air pollution by airplanes is through the continuous monitoring of O3 concentrations during different flight segments. The Measurement of Ozone and Water Vapor by Airbus In-Service Aircraft (MOZAIC) program, for instance, uses automatic instruments installed on long-range Airbus 340 aircraft to measure O3 and water vapour in the atmosphere. Similar instrumentation could be used for routine monitoring of O3 inside aircraft cabins, as high O3 concentrations can be encountered on flights, especially during late winter and early spring.
Furthermore, the impact of aircraft idling can be assessed through the development and application of global models that quantify the air quality impacts of aviation emissions. For example, the GEOS-Chem High-Performance chemistry-transport model uses a 50 km global resolution to provide a single, internally consistent global approach to quantifying the effects of aviation emissions. This model has helped estimate the number of premature mortalities attributable to aviation emissions, which varies across studies, with one source estimating 58,000 premature deaths per year.
Overall, assessing the impact of aircraft idling is crucial for developing environmental mitigation policies for the aviation industry and ensuring the safety and well-being of the public. By employing various models and monitoring techniques, we can better understand the health and environmental consequences of aircraft idling and work towards reducing its impact on air pollution.
Air Quality Monitoring: Measuring Pollutants Accurately
You may want to see also
Using nephelometers and piezoelectric balances
Nephelometers are instruments used to measure the distribution of particulate matter in gases, liquids, and ocean waters. They are also used to track the resuspension and dispersion of sediments.
A nephelometer or aerosol photometer measures the concentration of suspended particulates in a liquid or gas colloid. It employs a light beam (source beam) and a light detector set to one side (often at a 90-degree angle) of the source beam. Particle density is then a function of the light reflected into the detector from the particles. The amount of light reflected depends on the properties of the particles, such as their shape, color, and reflectivity.
To compensate for the different colors of dust, nephelometers are calibrated to a known particulate, and then environmental factors (k-factors) are used. The user determines the k-factor by running the nephelometer next to an air sampling pump and comparing the results.
In the context of air pollution measurement in airplanes, a nephelometer can be used to monitor the air that leaves the passenger cabin and is directed to the recirculation or exhaust system. It provides a continuous and online indication and recording of the mass concentration of fine particles, thus determining the potential exposure of passengers and cabin attendants to combustion byproducts.
The sensing zone of the nephelometer, when located in the air leaving the passenger cabin, can determine the highest particle concentration in the cabin. The output signals from the nephelometers should be connected to the aircraft's air-quality data recorder for analysis and correlation with reported health problems.
While a nephelometer can provide real-time data on particle concentrations, it cannot provide information on the chemical or biological composition of the particles. When such information is required for forensic evaluation of air-quality problems, it can be obtained from PM samples that are collected on filters during the flight and then analyzed using sensitive laboratory techniques.
Piezoelectric balances are not mentioned in the sources.
Radon Gas: A Silent Indoor Air Pollutant?
You may want to see also
Evaluating air quality near airports
A systematic literature review of peer-reviewed articles from 2000 to 2020 revealed that ultrafine particulate matter (UFP) is consistently elevated in and around airports. Additionally, high levels of particulate matter under 2.5 microns in diameter (PM2.5), black carbon, criteria pollutants, and polycyclic aromatic hydrocarbons were also found. These pollutants can have adverse health effects, including increased rates of premature death, pre-term births, decreased lung function, oxidative DNA damage, and childhood leukaemia.
Ozone (O3) is another critical factor in evaluating air quality near airports. O3-destroying catalysts are often used on high-altitude polar flights, but data from NASA in the 1970s showed that high O3 levels can also be encountered on flights at lower latitudes, particularly during late winter and early spring. Continuous O3 monitoring is necessary to understand the full extent of its presence in aircraft cabins and the ambient air. The MOZAIC program, which uses automatic instruments installed on long-range Airbus aircraft, is one such initiative aimed at measuring O3 and water vapour in the atmosphere.
To address the impact of aviation emissions on air quality, the EPA has proposed and finalized greenhouse gas (GHG) emission standards for new commercial airplanes and their engines. These standards align with the international CO2 standards set by the International Civil Aviation Organization (ICAO) in 2017. By regulating emissions from aircraft, these standards aim to mitigate their contribution to air pollution and protect public health and welfare.
Overall, evaluating air quality near airports involves assessing the levels of various pollutants, including UFP, PM2.5, black carbon, and ozone, and understanding their potential health effects on nearby populations. This knowledge is crucial for developing effective strategies to mitigate the impact of commercial aircraft activity on air quality and public health.
Air Pollutants: Harmful Impact on Human Health
You may want to see also
Frequently asked questions
Aircraft idling and taxiing are major sources of airport air pollution. Aircraft engines emit pollutants such as carbon monoxide, hydrocarbons, and particles.
Air pollution from civil aviation emissions has been linked to adverse health effects, including increased rates of premature death, pre-term births, decreased lung function, oxidative DNA damage, and childhood leukemia.
Air pollution from airplanes can be measured using portable instruments to assess ETS concentrations on commercial flights. A hand-held nephelometer and piezoelectric balance, for example, can be used to measure the mass concentration of suspended particulate matter.
The EPA has finalized greenhouse gas (GHG) emission standards for certain new commercial airplanes, including all large passenger jets. These standards align with the international carbon dioxide (CO2) standards set by the International Civil Aviation Organization (ICAO) in 2017.