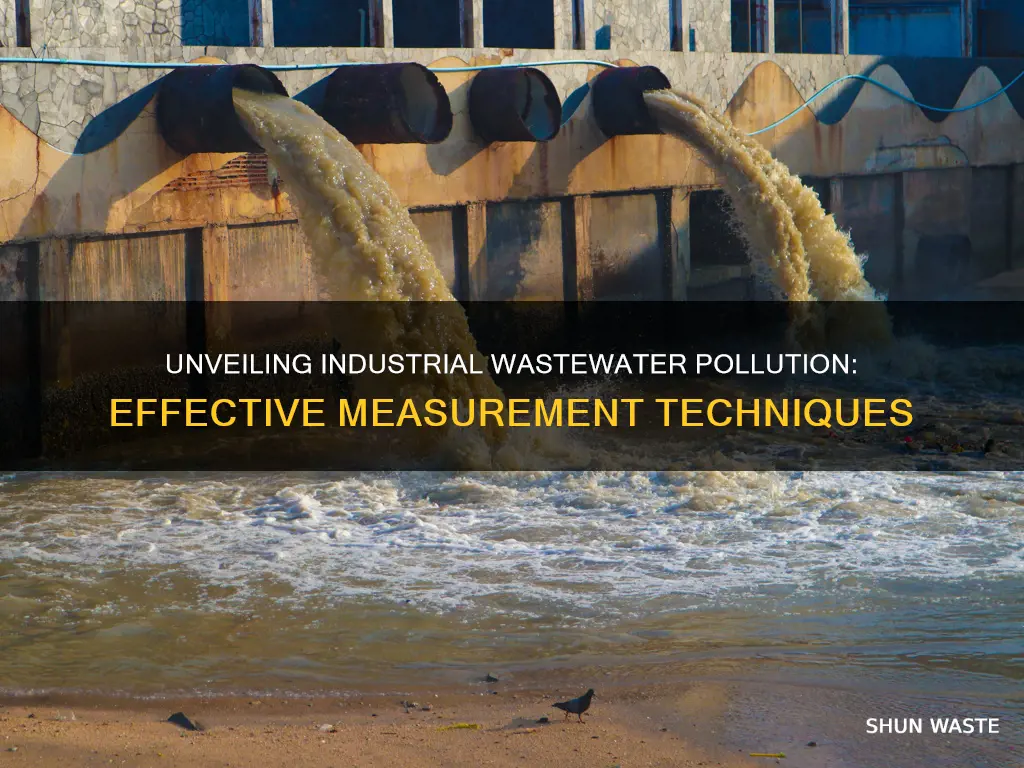
Industrial wastewater pollution is a critical environmental concern, and accurate measurement is essential for effective management and regulation. This paragraph introduces the topic by highlighting the importance of assessing and monitoring industrial wastewater to ensure compliance with environmental standards and protect water resources. It mentions that various methods and technologies are available to measure and analyze pollutants, including physical, chemical, and biological testing, as well as advanced monitoring systems. By understanding and implementing these measurement techniques, industries can contribute to sustainable practices and minimize their environmental impact.
What You'll Learn
- Physical Measurements: Use turbidity meters and pH probes for initial assessments
- Chemical Analysis: Employ titration, chromatography, and spectroscopy for detailed pollutant identification
- Biological Testing: Monitor microbial activity and biodiversity to assess ecosystem health
- Radiometric Methods: Utilize gamma-ray spectroscopy for trace element detection in wastewater
- Remote Sensing: Satellite imagery and drones aid in large-scale pollution monitoring and mapping
Physical Measurements: Use turbidity meters and pH probes for initial assessments
When assessing industrial wastewater pollution, physical measurements are a crucial initial step to gain a comprehensive understanding of the water's quality. One of the primary tools for this assessment is the use of turbidity meters. Turbidity refers to the cloudiness or opacity of water, which is often caused by suspended particles such as sediments, oils, or other contaminants. These meters work by measuring the amount of light that passes through the water and calculating the turbidity based on the light scattering. By taking readings at various points in the wastewater stream, you can identify areas of higher turbidity, which may indicate the presence of pollutants or areas where treatment is required. This non-invasive method provides a quick and efficient way to gauge the overall health of the water and pinpoint potential sources of contamination.
In conjunction with turbidity measurements, pH probes are essential tools for initial assessments. pH is a measure of the acidity or alkalinity of the water, which is a critical factor in determining its suitability for various purposes. Industrial wastewater often has a pH that deviates from the neutral 7, which can be an indicator of chemical pollutants. pH probes are designed to measure the hydrogen-ion concentration in the water, providing a precise reading of its acidity or basicity. By monitoring pH levels, you can assess whether the wastewater is corrosive, scaling, or potentially harmful to the environment. This information is vital for selecting appropriate treatment methods and ensuring compliance with regulatory standards.
The process of using these physical measurement tools is straightforward. Turbidity meters are typically placed in the wastewater stream, often at different stages of the treatment process, to capture a representative sample. These meters can be portable or fixed, allowing for both on-site and laboratory measurements. Similarly, pH probes are inserted into the water to measure its pH. It is important to calibrate these instruments regularly to ensure accurate readings. By taking multiple samples and measurements, you can create a comprehensive profile of the wastewater's physical properties, which is the first step towards effective pollution control and treatment.
Initial assessments using turbidity meters and pH probes provide valuable insights into the nature of industrial wastewater pollution. These measurements offer a quick and non-invasive way to identify potential issues, such as high turbidity indicating sediment or oil contamination, or a low pH suggesting acidic chemicals. This information is crucial for making informed decisions about treatment strategies and ensuring that the wastewater meets the required standards before being discharged or reused. Furthermore, these physical measurements can help in optimizing the overall treatment process, reducing costs, and minimizing environmental impact.
In summary, the use of turbidity meters and pH probes is a fundamental and practical approach to measuring industrial wastewater pollution. These tools enable environmental scientists and engineers to make informed decisions about pollution control and treatment, ensuring that the water is safe and suitable for its intended purpose. By incorporating these physical measurements into the initial assessment, industries can take proactive steps towards maintaining water quality and adhering to environmental regulations.
Water's Toxic Embrace: The Plight of Birds
You may want to see also
Chemical Analysis: Employ titration, chromatography, and spectroscopy for detailed pollutant identification
Chemical analysis plays a crucial role in assessing and understanding the composition of industrial wastewater, allowing for the identification and quantification of various pollutants. This process involves employing a range of sophisticated techniques to gain detailed insights into the chemical properties and characteristics of the water samples.
One of the fundamental methods used in chemical analysis is titration. This technique involves adding a known reagent to the wastewater sample until a specific endpoint is reached, often indicated by a color change or a measurable property. For instance, acid-base titration can determine the pH level and the concentration of acids or bases in the water. This is particularly important as it helps identify the presence of corrosive or alkaline substances that may affect the environment and equipment. Additionally, redox titration can measure the levels of oxidizing or reducing agents, providing insights into the water's chemical reactivity.
Chromatography is another powerful tool in the chemical analysis of industrial wastewater. This technique separates and analyzes the individual components of a mixture, allowing for the identification and quantification of various pollutants. Liquid chromatography (LC) and gas chromatography (GC) are commonly used methods. LC can separate and detect organic compounds, heavy metals, and inorganic ions, making it ideal for identifying a wide range of contaminants. GC, on the other hand, is suitable for volatile organic compounds and can provide detailed information about the chemical composition of the wastewater. These chromatography techniques often use advanced detection systems, such as mass spectrometry, to ensure accurate and precise pollutant identification.
Spectroscopy is a non-destructive analytical technique that provides valuable information about the molecular structure and composition of wastewater samples. Techniques like atomic absorption spectroscopy (AAS) and inductively coupled plasma mass spectrometry (ICP-MS) are employed to measure the concentration of various elements, including heavy metals, in the water. These methods offer high sensitivity and selectivity, allowing for the detection of even trace amounts of pollutants. Additionally, infrared (IR) and Raman spectroscopy can identify organic compounds and provide information about their functional groups, aiding in the characterization of complex mixtures.
By combining titration, chromatography, and spectroscopy, a comprehensive chemical analysis of industrial wastewater can be achieved. These techniques enable the identification of a wide range of pollutants, including organic compounds, heavy metals, nutrients, and other contaminants. The data obtained from these analyses is essential for understanding the environmental impact of industrial processes, compliance with regulatory standards, and the development of effective treatment strategies to mitigate water pollution. Furthermore, this detailed information can guide the implementation of sustainable practices to minimize the release of harmful substances into the water environment.
Unveiling the Essence: Understanding Water Sources
You may want to see also
Biological Testing: Monitor microbial activity and biodiversity to assess ecosystem health
Biological testing is a crucial method for assessing the health of aquatic ecosystems and understanding the impact of industrial wastewater pollution. This approach focuses on examining the microbial communities and their activities within the water body, providing valuable insights into the overall environmental quality. By monitoring microbial activity and biodiversity, scientists and environmental professionals can make informed decisions regarding pollution levels and potential ecological risks.
The process begins with collecting water samples from the industrial site and nearby control areas, ensuring a representative and diverse set of data. These samples are then analyzed for their microbial content, which can be achieved through various techniques. One common method is the use of culture-based approaches, where specific media and conditions are provided to encourage the growth of different microorganisms. By identifying and counting the colonies of various bacteria, fungi, and other microbes, researchers can determine the diversity and abundance of these organisms. This information is vital as it indicates the overall health of the ecosystem and the potential for biological degradation of pollutants.
Advanced molecular biology techniques, such as DNA sequencing and gene expression analysis, offer more comprehensive insights. These methods allow for the identification of specific microbial species and their metabolic activities, even in low-abundance scenarios. By studying the genetic makeup of the microbial community, scientists can assess the presence of pollutant-degrading bacteria and their efficiency in breaking down industrial waste. For instance, certain bacteria can metabolize heavy metals, organic compounds, or nutrients, providing a natural treatment mechanism for polluted water.
In addition to microbial activity, biodiversity assessment is essential. This involves evaluating the variety and abundance of different species within the ecosystem. High biodiversity often indicates a healthy and resilient environment, capable of withstanding and recovering from pollution. Conversely, a decline in biodiversity may suggest the presence of toxic substances or other stressors affecting the microbial community. By comparing the biodiversity of polluted and control sites, researchers can establish baseline data and monitor changes over time, helping to identify the ecological impact of industrial activities.
The results of biological testing provide a holistic view of wastewater pollution. They can indicate the presence of harmful substances, the extent of pollution, and the potential risks to aquatic life and human health. Furthermore, this data aids in the development of effective remediation strategies, such as implementing biological treatment processes or restoring natural habitats to enhance ecosystem resilience. Regular monitoring and biological testing are essential tools for industries to ensure compliance with environmental regulations and contribute to sustainable water management practices.
Unveiling Water's Secrets: A Guide to Identifying Hidden Pollutants
You may want to see also
Radiometric Methods: Utilize gamma-ray spectroscopy for trace element detection in wastewater
Radiometric methods, particularly gamma-ray spectroscopy, offer a powerful tool for trace element detection in industrial wastewater, providing a non-destructive and highly sensitive approach to assessing water quality. This technique is valuable for identifying and quantifying trace metals and other radioactive elements that may be present in wastewater, even at very low concentrations.
The process begins with the collection of a water sample, which is then carefully prepared to ensure it is free from contaminants that could interfere with the analysis. The sample is placed in a gamma-ray spectrometer, a specialized instrument designed to detect and measure the energy levels of gamma rays emitted by the elements present in the water. Each element has a unique energy signature, allowing for specific identification. For instance, cesium-137 and cobalt-60 are commonly detected in industrial settings due to their radioactive decay processes.
Gamma-ray spectroscopy provides a rapid and accurate assessment of the sample. The spectrometer records the energy and intensity of the gamma rays, which are then analyzed to determine the concentration of trace elements. This method is highly sensitive, capable of detecting elements at parts per billion (ppb) or even parts per trillion (ppt) levels, far below the concentrations that might be visible through other analytical techniques. This sensitivity is crucial for industries, as it allows for the early detection of potential pollution sources and the implementation of corrective measures before environmental damage occurs.
One of the key advantages of radiometric methods is their ability to provide real-time data. The spectrometer can continuously monitor the sample, offering immediate feedback on the water's chemical composition. This real-time analysis is particularly useful for process control in industrial settings, where rapid adjustments can be made to prevent contamination. Additionally, the non-destructive nature of gamma-ray spectroscopy means that the water sample remains intact, allowing for further testing or release if the analysis confirms the water's safety.
In summary, radiometric methods, specifically gamma-ray spectroscopy, are an invaluable tool for trace element detection in industrial wastewater. This technique offers high sensitivity, rapid analysis, and the ability to identify a wide range of elements, making it an essential component of modern wastewater management and environmental monitoring systems. By utilizing this method, industries can ensure compliance with environmental regulations and maintain the health of their water resources.
Government Strategies: Tackling Water Pollution Head-On
You may want to see also
Remote Sensing: Satellite imagery and drones aid in large-scale pollution monitoring and mapping
Remote sensing technology, including satellite imagery and drones, has revolutionized the way we monitor and map environmental changes, especially in the context of large-scale pollution. These tools provide a unique perspective and an extensive view of the Earth's surface, making them invaluable for assessing and managing industrial wastewater pollution.
Satellite imagery offers a powerful means to detect and analyze pollution on a regional or even global scale. High-resolution satellites can capture detailed images of water bodies, allowing scientists and environmental experts to identify and quantify pollutants. For instance, by analyzing spectral signatures, satellites can detect and differentiate between various pollutants, such as oil spills, industrial effluents, or agricultural runoff. This capability is particularly useful for monitoring coastal areas, rivers, and lakes where industrial activities are prevalent.
The use of drones, or Unmanned Aerial Vehicles (UAVs), complements satellite data by providing a more localized and dynamic perspective. Equipped with high-resolution cameras and sensors, drones can capture aerial images and videos of specific areas, offering a bird's-eye view of pollution sources and their impact. Drones can access hard-to-reach locations, providing valuable insights into industrial sites, treatment plants, or even illegal dumping activities. Real-time data collection by drones enables rapid response to pollution incidents, ensuring timely mitigation measures.
In the context of wastewater pollution, remote sensing can identify and map sources of contamination, such as industrial pipes, sewage outfalls, or agricultural drainage systems. By analyzing temporal changes in water quality, satellites can detect anomalies and potential pollution hotspots. For example, a sudden change in water color or temperature could indicate an industrial leak or natural disaster. Drones can further verify these anomalies by capturing high-resolution images, aiding in the identification of pollution sources and their extent.
Furthermore, the integration of remote sensing data with geographic information systems (GIS) allows for the creation of comprehensive pollution maps. These maps can visualize pollution levels, sources, and trends over time, providing valuable information for environmental agencies and policymakers. By combining satellite and drone data with ground-based measurements, a more holistic understanding of wastewater pollution can be achieved, enabling better-informed decisions for pollution control and management.
In summary, remote sensing technologies, including satellite imagery and drones, play a crucial role in large-scale pollution monitoring and mapping. They provide a unique and comprehensive view of the Earth's surface, enabling the detection, analysis, and visualization of industrial wastewater pollution. With their ability to access diverse environments and capture real-time data, these technologies contribute significantly to environmental protection and sustainable management practices.
Nature's Hidden Polluters: Unveiling the Water Contaminants
You may want to see also
Frequently asked questions
There are several techniques to assess water quality, including physical, chemical, and biological methods. Physical measurements involve assessing parameters like temperature, pH, and turbidity. Chemical analysis determines the concentration of pollutants such as heavy metals, nutrients, and organic compounds. Biological methods utilize microorganisms or plant responses to detect contaminants.
Heavy metal analysis in wastewater often employs atomic absorption spectroscopy (AAS) or inductively coupled plasma mass spectrometry (ICP-MS). These techniques provide highly sensitive and accurate measurements of metal concentrations, allowing for the detection of even trace amounts.
Dissolved oxygen levels are crucial indicators of water quality and aquatic life. In wastewater treatment, DO measurement helps assess the efficiency of biological processes. Low DO levels may indicate the presence of organic pollutants, while high DO can suggest efficient oxygen transfer and treatment process performance.
BOD is a measure of the amount of oxygen required by microorganisms to decompose organic matter in water. It is typically measured by incubating a water sample with an indicator and measuring the oxygen consumption over a specific period. This test provides insights into the organic pollution level in the wastewater.
Online monitoring systems offer continuous data collection and analysis for efficient pollution control. These systems may include sensors for pH, conductivity, dissolved oxygen, and turbidity. Advanced systems can also measure specific pollutants like ammonia, nitrate, and total phosphorus, providing real-time insights into wastewater quality.