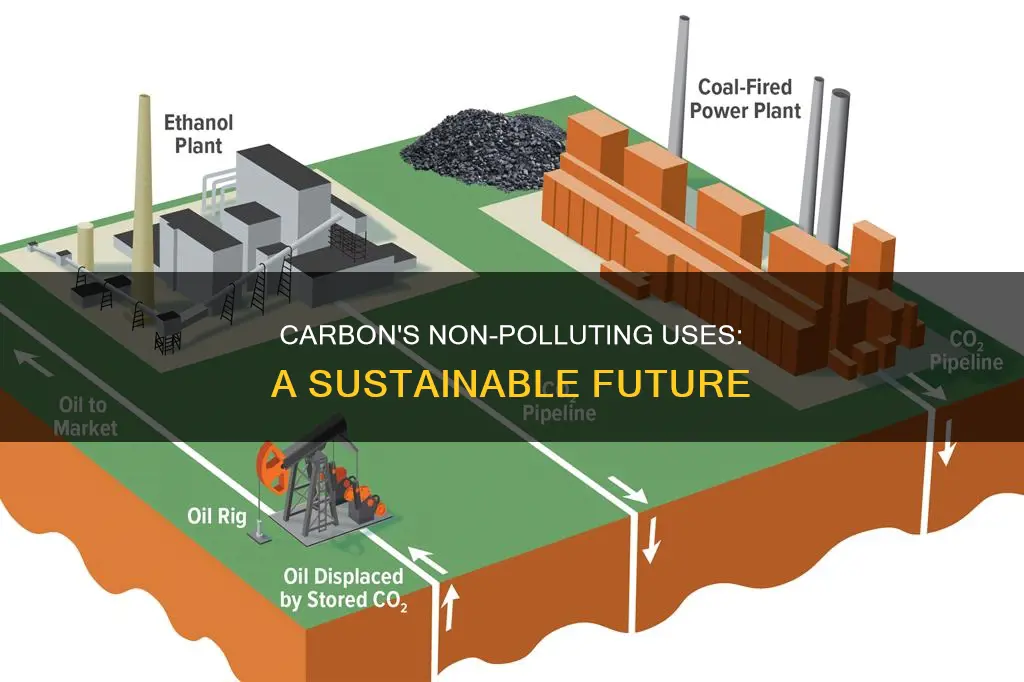
Carbon dioxide (CO2) is a greenhouse gas and a major contributor to global warming. The large-scale burning of fossil fuels has led to a dramatic increase in its concentration in the Earth's atmosphere, causing a rise in global temperatures. While CO2 molecules can be broken down and reused, the current methods for doing so are energy-intensive and often involve burning more fossil fuels, which emit additional greenhouse gases. However, recent innovations in technology have offered potential solutions to this issue. Researchers at the Massachusetts Institute of Technology (MIT) have developed a system that converts CO2 into a safe, storable salt that can be used to generate electricity. This process addresses the challenge of capturing and storing carbon dioxide while also creating a usable product. Additionally, scientists are exploring low-carbon methods for splitting CO2 molecules, such as using solar energy to power chemical reactors that produce synthetic fuels. These advancements in carbon capture and utilization technologies present promising avenues for reducing carbon emissions and mitigating the impacts of climate change.
What You'll Learn
Carbon capture and storage
The process involves capturing CO2 emissions from industrial processes such as steel and cement production, or from burning fossil fuels in power generation. The CO2 is then transported via ship or pipeline and stored deep underground in geological formations. Possible storage sites include saline aquifers or depleted oil and gas reservoirs, typically at least 0.62 miles (1km) underground.
CCS has been in operation since 1972 in the US, where several natural gas plants in Texas have captured and stored more than 200 million tons of CO2 underground. As of 2024, CCS was in operation at 44 plants worldwide, capturing about one-thousandth of global carbon dioxide emissions. The effectiveness of CCS in reducing carbon emissions depends on the plant's capture efficiency, the additional energy used for CCS, leakage, and business and technical issues.
CCS could play a critical but limited role in reducing greenhouse gas emissions. However, other emission-reduction options such as solar and wind energy, electrification, and public transit are less expensive and more effective at reducing air pollution. CCS is envisioned to be most useful in specific niches, such as heavy industry and plant retrofits.
There are some concerns about CCS, including the fact that plants with CCS require more energy to operate, leading to increased pollution from extracting and transporting fuel. Additionally, CCS facilities use more energy and water than those without CCS, resulting in "upstream" environmental problems. There is also a risk of CO2 leakage from storage sites, which could be dangerous to nearby communities.
Overall, while CCS has the potential to play a role in reducing carbon dioxide emissions, it is not a silver bullet and should be combined with other emission-reduction strategies.
Air Pollutants: A Journey from Lungs to Blood
You may want to see also
Carbon-to-fuel conversion
The process of converting carbon dioxide (CO2) into fuel offers a promising solution to minimise global warming and meet the world's increasing energy demand. This approach aims to transform a significant contributor to climate change into a valuable resource.
Carbon Capture and Conversion:
The first step in carbon-to-fuel conversion is capturing CO2 from power plant emissions or even the open air. This can be achieved through various methods, such as using manufactured CaO sorbent material derived from seashells or eggshells, which effectively attract and capture CO2.
Chemical Conversion:
Once captured, CO2 undergoes chemical conversion to transform it into fuel. One method involves a two-stage process: first, CO2 is chemically captured and turned into solid calcium carbonate; then, this material is heated to release CO2 and convert it into a fuel feedstock like carbon monoxide. However, this approach has low efficiency, typically converting less than 20% of the gaseous CO2.
An alternative chemical conversion process, developed by researchers at MIT and Harvard University, achieves a conversion rate of over 90%. This innovative technique first converts CO2 into an intermediate form, liquid metal bicarbonate, and then uses an electrolyzer to convert it into liquid potassium or sodium formate. The resulting highly concentrated formate solution can be dried to produce a stable powder that can be stored and used as fuel when needed.
Biological Conversion:
Biological conversion methods leverage agriculture, fermentation, and genetic engineering to convert CO2 into products using microorganisms, including algal systems and non-photosynthetic microbes. For example, algae can be used to convert CO2 into lipids, carbohydrates, and proteins, which can then be processed into biofuels.
Catalytic Conversion:
Catalytic conversion approaches employ thermochemical, electrochemical, photochemical, reactive capture, and plasma-assisted techniques to transform CO2 into synthetic fuels, chemicals, and other products. One such approach, developed by researchers at the Massachusetts Institute of Technology (MIT), converts CO2 into a safe and storable salt that can power a fuel cell to generate electricity.
Benefits and Challenges:
The conversion of CO2 into fuel provides a way to reduce global warming by decreasing the concentration of this greenhouse gas in the atmosphere. It also addresses the issue of declining fossil fuel reserves in the face of rising energy demand. However, challenges remain, including the stability and inertness of CO2, which make it difficult to obtain value-added fuels through chemical conversion processes.
Overall, the carbon-to-fuel conversion process holds great potential for mitigating climate change and meeting energy demands while also creating economically valuable and environmentally responsible products.
Air Pollution's Link to Emphysema: A Health Warning
You may want to see also
Carbon monoxide and oxygen separation
Carbon monoxide and oxygen can be separated from carbon dioxide through a process called direct air capture (DAC). DAC involves using machines to suck in air and chemically bind it with a non-toxic solution to separate out the carbon dioxide.
However, DAC is an energy-intensive process, and the energy required to run DAC machines as a primary climate solution is equivalent to the current annual energy needs of Japan, China, the US, and the EU combined. Therefore, for DAC to be a viable climate solution, the energy used to power the machines would need to come from low- or no-carbon sources such as wind, solar, and nuclear power.
Another method to separate carbon monoxide and oxygen from carbon dioxide is through carbon capture, utilization, and storage (CCUS). CCUS can be used to capture carbon dioxide from major land-based sources like factories or power plants.
Once carbon dioxide is captured through DAC or CCUS, it can be split into carbon monoxide and oxygen. However, the current process of splitting carbon dioxide requires high temperatures of 1300° F/700° C or more, which is energy-intensive and often accomplished by burning fuels that emit greenhouse gases.
Scientists are exploring low-carbon methods of splitting carbon dioxide, such as using solar energy to power thermal chemical reactors. Overall, the development of energy-efficient carbon monoxide separation processes is crucial for both industrial and environmental purposes.
Pumping Pollution Underground: A Viable Solution?
You may want to see also
Carbon-neutral energy sources
Synthetic Fuels
Synthetic fuels are made by chemically hydrogenating carbon dioxide. The carbon dioxide used to make synthetic fuels can be captured from the air, recycled from power plant flue exhaust gas, or derived from carbonic acid in seawater. Common examples include ammonia and methane, although more complex hydrocarbons such as gasoline and jet fuel have also been artificially synthesized. The energy used in the production of synthetic fuels must itself be carbon-neutral or emissions-free, like renewable energy or nuclear energy.
Biofuels
Biofuels are produced using natural CO2-consuming processes like photosynthesis. Bioenergy produced from biomass is sometimes called a carbon-neutral energy source because the same amount of carbon released when the biomass is burned is sequestered again when the crop or forest is regrown. However, there are emissions associated with producing biomass, such as from fossil fuels used in cultivation, harvest, processing, transport, and the manufacture and construction of fuel conversion technology. Nevertheless, the greenhouse impact of bioenergy is much lower than that of fossil fuel energy sources.
Other Carbon-Neutral Energy Sources
Other carbon-neutral energy sources include nuclear, geothermal, and solar thermal power. Zero-carbon fuels such as hydrogen and ammonia can also be used as alternatives to traditional fuel combustion for industrial applications.
Air Pollution's Soil Loss: A Troubling Connection
You may want to see also
Carbon utilisation and storage
Carbon capture, utilisation, and storage (CCUS) is a way of reducing carbon dioxide (CO2) emissions, which is key to tackling global warming. The process involves three steps: capturing CO2 produced by power generation or industrial activity, transporting it, and storing it permanently underground.
CCUS can be retrofitted to existing power and industrial plants, allowing them to continue operating while reducing emissions. It is particularly useful for heavy industries like cement, steel, and chemicals, which are challenging to decarbonise. CCUS enables the production of low-carbon hydrogen, supporting the decarbonisation of other sectors such as industry, trucks, and ships. Additionally, CCUS can remove CO2 directly from the air, addressing emissions that are hard to abate.
There are currently around 45 commercial CCUS facilities in operation globally, with a total annual capture capacity of over 50 million tonnes of CO2. While CCUS deployment has gained momentum, it still falls short of what is required to achieve net-zero emissions.
CCUS involves capturing CO2 from large point sources, such as power generation or industrial facilities using fossil fuels or biomass as fuel. The captured CO2 is then compressed and transported via pipeline, ship, rail, or truck. It can be utilised in various applications, such as converting it into plastics, concrete, or biofuel. Alternatively, it can be injected into deep geological formations, such as depleted oil and gas reservoirs or saline aquifers, for permanent storage.
CCUS has been in operation since the 1970s, with natural gas plants in Texas capturing and storing CO2 underground. However, its potential to mitigate climate change has not been fully realised due to slow deployment and limited impact on global CO2 emissions. Nevertheless, CCUS remains a critical component in the transition to clean energy and achieving net-zero emissions targets.
Soil Pollution: Atmospheric Deposition's Impact on Earth's Health
You may want to see also
Frequently asked questions
Carbon dioxide can be converted into a safe, storable salt that can be used to run a fuel cell to make electricity. This process involves using a non-toxic solution to separate out the CO2, and then converting it into a formate salt that can be used to power a fuel cell.
Using carbon dioxide in this way can help to reduce the amount of CO2 in the atmosphere, which is a major contributor to global warming. It can also create a financial incentive for capturing and utilising CO2, as the resulting salt can be used to create marketable products.
Yes, carbon dioxide can be captured and stored underground, or used to create synthetic fuels and building supplies. Additionally, scientists are exploring low-carbon ways of splitting CO2 molecules to create cleaner high-energy fuels.